Generate a hypothesis in the research field of Materials Science.
Hypothesis: The incorporation of graphene nanosheets into polymer-based nanocomposites can significantly enhance the mechanical, thermal, and electrical properties of the resulting material, leading to the development of lightweight, high-performance materials for various applications, such as aerospace, automotive, and electronics industries.
Suggest a suitable experimental design to test the hypothesis.
Experimental Design for Testing the Hypothesis:
-
Objective: To investigate the effect of incorporating graphene nanosheets into polymer-based nanocomposites on their mechanical, thermal, and electrical properties.
-
Materials:
- Graphene nanosheets
- Polymer matrix (e.g., epoxy, polyethylene, or polystyrene)
- Solvents and dispersing agents
-
Appropriate equipment for composite fabrication (e.g., ultrasonicator, mechanical stirrer, hot press)
-
Methods:
-
Sample Preparation:
- Prepare a series of polymer-based nanocomposites with varying concentrations of graphene nanosheets (e.g., 0.1, 0.5, 1, 2, and 5 wt%).
- Disperse the graphene nanosheets in a suitable solvent using ultrasonication to ensure uniform dispersion.
- Mix the dispersed graphene nanosheets with the polymer matrix using a mechanical stirrer.
- Remove the solvent and fabricate the nanocomposite samples using a hot press or other appropriate methods.
-
Characterization:
- Perform scanning electron microscopy (SEM) and transmission electron microscopy (TEM) to analyze the dispersion and distribution of graphene nanosheets within the polymer matrix.
- Conduct X-ray diffraction (XRD) and Raman spectroscopy to investigate the structural changes in the nanocomposites.
-
Property Evaluation:
- Mechanical properties: Measure the tensile strength, modulus, and elongation at break using a universal testing machine (UTM) according to standard test methods (e.g., ASTM D638).
- Thermal properties: Analyze the thermal stability and glass transition temperature (Tg) using thermogravimetric analysis (TGA) and differential scanning calorimetry (DSC), respectively.
- Electrical properties: Measure the electrical conductivity of the nanocomposites using a four-point probe method or other suitable techniques.
-
Data Analysis:
- Analyze the obtained data to determine the correlation between the concentration of graphene nanosheets and the mechanical, thermal, and electrical properties of the nanocomposites.
-
Investigate the structure-property relationships and identify the optimal concentration of graphene nanosheets for achieving the desired properties.
-
Conclusion:
- Summarize the findings and discuss the potential applications of the developed graphene-polymer nanocomposites in various industries, such as aerospace, automotive, and electronics.
Predict possible outcomes of the experiment.
Possible Outcomes of the Experiment:
-
Improved mechanical properties: The incorporation of graphene nanosheets into the polymer matrix may result in enhanced tensile strength, modulus, and elongation at break. This improvement could be attributed to the strong interfacial bonding between the graphene nanosheets and the polymer matrix, as well as the reinforcement effect provided by the nanosheets.
-
Enhanced thermal properties: The addition of graphene nanosheets may lead to increased thermal stability and a higher glass transition temperature (Tg) of the nanocomposites. This could be due to the excellent thermal conductivity of graphene, which facilitates heat dissipation and reduces thermal degradation of the polymer matrix.
-
Increased electrical conductivity: The electrical conductivity of the nanocomposites may increase with the addition of graphene nanosheets, as graphene is known for its exceptional electrical conductivity. The percolation threshold, where a continuous conductive network is formed within the polymer matrix, may be observed at a certain concentration of graphene nanosheets.
-
Optimal concentration of graphene nanosheets: There may be an optimal concentration of graphene nanosheets that yields the best combination of mechanical, thermal, and electrical properties. Beyond this concentration, the properties may plateau or even deteriorate due to issues such as agglomeration of nanosheets or increased viscosity of the composite, making it difficult to process.
-
Structure-property relationships: The experiment may reveal correlations between the dispersion and distribution of graphene nanosheets within the polymer matrix and the resulting properties of the nanocomposites. A uniform dispersion and distribution of nanosheets could lead to more significant improvements in the properties.
-
Potential applications: Based on the observed improvements in mechanical, thermal, and electrical properties, the developed graphene-polymer nanocomposites may show promise for various applications in industries such as aerospace, automotive, and electronics. Examples include lightweight structural components, thermal management systems, and conductive materials for electronic devices.
Discuss the implications of the results for the original hypothesis.
The implications of the experimental results for the original hypothesis can be discussed as follows:
-
Confirmation of the hypothesis: If the results show significant improvements in the mechanical, thermal, and electrical properties of the polymer-based nanocomposites upon the incorporation of graphene nanosheets, it would confirm the original hypothesis. This would demonstrate the potential of graphene-polymer nanocomposites as high-performance materials for various applications in aerospace, automotive, and electronics industries.
-
Optimal concentration of graphene nanosheets: The experiment may reveal an optimal concentration of graphene nanosheets that provides the best combination of properties. This information would be valuable for tailoring the properties of the nanocomposites to meet specific application requirements and for guiding future research on graphene-polymer nanocomposites.
-
Structure-property relationships: Understanding the correlations between the dispersion and distribution of graphene nanosheets within the polymer matrix and the resulting properties of the nanocomposites would provide insights into the underlying mechanisms responsible for the observed improvements. This knowledge could be used to optimize the fabrication process and further enhance the properties of the nanocomposites.
-
Potential limitations and challenges: The experiment may also reveal potential limitations and challenges associated with the incorporation of graphene nanosheets into polymer-based nanocomposites, such as agglomeration of nanosheets, increased viscosity, or processing difficulties. Identifying these challenges would help researchers develop strategies to overcome them and improve the overall performance of the nanocomposites.
-
Future research directions: The results of the experiment could open up new avenues for further research in the field of materials science. For example, researchers could explore the use of different types of polymers, graphene derivatives, or other nanofillers to develop novel nanocomposites with tailored properties. Additionally, the potential applications of the developed graphene-polymer nanocomposites could be investigated in more detail, including their performance under real-world conditions and their long-term durability.
In summary, the experimental results would have significant implications for the original hypothesis, either confirming or refuting it. The findings would contribute to the understanding of graphene-polymer nanocomposites, their structure-property relationships, and their potential applications in various industries. Furthermore, the results would help identify potential challenges and limitations, guiding future research in this field.
Propose a follow-up study based on the findings.
Follow-up Study:
Title: Investigation of the Synergistic Effects of Graphene Nanosheets and Carbon Nanotubes on the Mechanical, Thermal, and Electrical Properties of Polymer-Based Nanocomposites
Objective: To explore the combined effects of graphene nanosheets and carbon nanotubes (CNTs) as hybrid nanofillers on the mechanical, thermal, and electrical properties of polymer-based nanocomposites, aiming to further enhance their performance and broaden their potential applications.
Materials: - Graphene nanosheets - Carbon nanotubes (single-walled or multi-walled) - Polymer matrix (e.g., epoxy, polyethylene, or polystyrene) - Solvents and dispersing agents - Appropriate equipment for composite fabrication (e.g., ultrasonicator, mechanical stirrer, hot press)
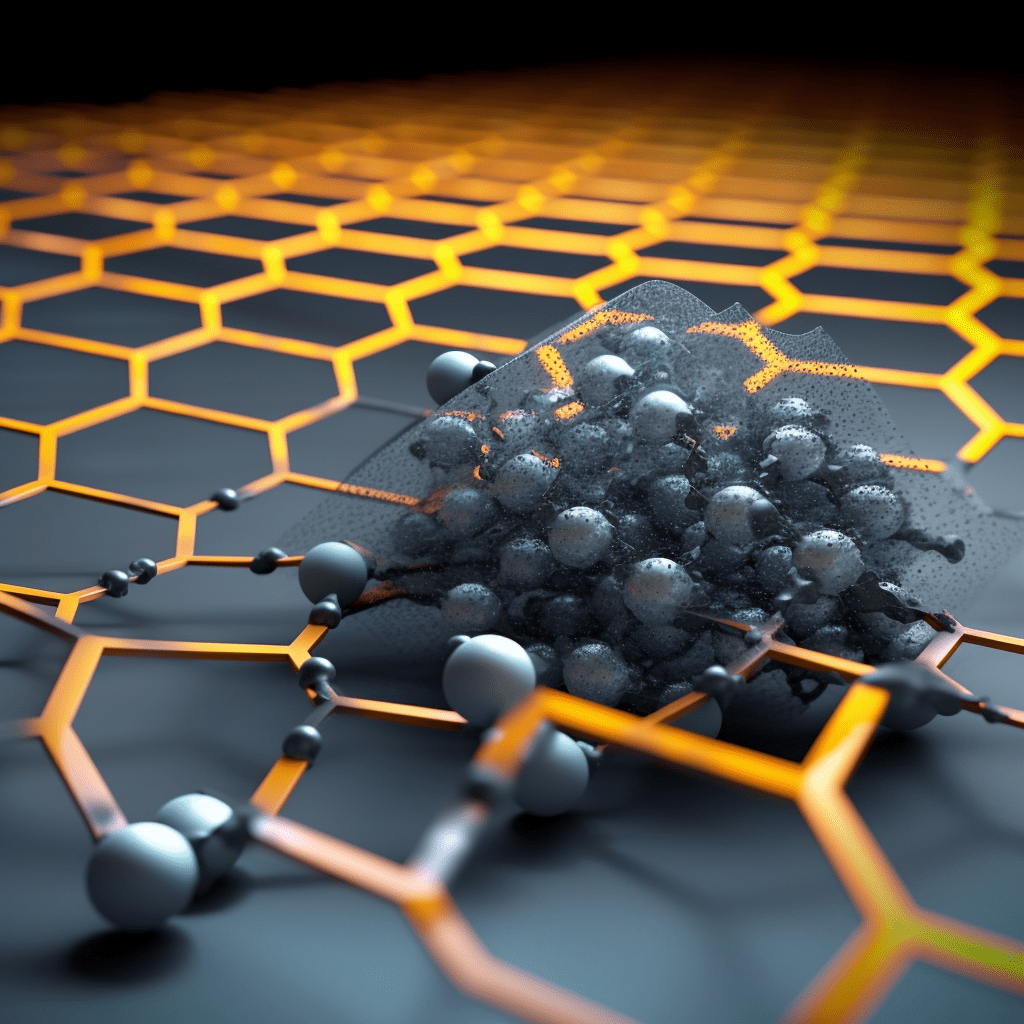{: .wrap-image}Methods:
- Sample Preparation:
- Prepare a series of polymer-based nanocomposites with varying concentrations and ratios of graphene nanosheets and carbon nanotubes (e.g., 1:0, 1:1, 1:2, 2:1, and 0:1).
- Disperse the graphene nanosheets and carbon nanotubes separately in a suitable solvent using ultrasonication to ensure uniform dispersion.
- Mix the dispersed graphene nanosheets and carbon nanotubes with the polymer matrix using a mechanical stirrer.
-
Remove the solvent and fabricate the nanocomposite samples using a hot press or other appropriate methods.
-
Characterization:
- Perform SEM and TEM to analyze the dispersion, distribution, and interaction of graphene nanosheets and carbon nanotubes within the polymer matrix.
-
Conduct XRD and Raman spectroscopy to investigate the structural changes in the nanocomposites.
-
Property Evaluation:
- Mechanical properties: Measure the tensile strength, modulus, and elongation at break using a UTM according to standard test methods (e.g., ASTM D638).
- Thermal properties: Analyze the thermal stability and glass transition temperature (Tg) using TGA and DSC, respectively.
-
Electrical properties: Measure the electrical conductivity of the nanocomposites using a four-point probe method or other suitable techniques.
-
Data Analysis:
- Analyze the obtained data to determine the correlation between the concentrations and ratios of graphene nanosheets and carbon nanotubes and the mechanical, thermal, and electrical properties of the nanocomposites.
-
Investigate the structure-property relationships and identify the optimal combination of graphene nanosheets and carbon nanotubes for achieving the desired properties.
-
Conclusion:
- Summarize the findings and discuss the potential applications of the developed hybrid graphene-CNT-polymer nanocomposites in various industries, such as aerospace, automotive, and electronics.
Rationale: The follow-up study aims to build upon the findings of the original experiment by exploring the synergistic effects of graphene nanosheets and carbon nanotubes as hybrid nanofillers in polymer-based nanocomposites. This approach could potentially lead to further improvements in the mechanical, thermal, and electrical properties of the nanocomposites, expanding their range of applications and providing new insights into the structure-property relationships of these materials.
Explore the potential real-world applications of the discovery.
The potential real-world applications of the graphene-polymer nanocomposites with enhanced mechanical, thermal, and electrical properties can be categorized into several industries:
- Aerospace:
- Lightweight structural components: The improved mechanical properties of the nanocomposites can be utilized to develop lightweight and strong components for aircraft, reducing overall weight and fuel consumption.
- Thermal management systems: The enhanced thermal properties can be employed in thermal management systems for heat dissipation in avionics and other electronic components.
-
Electromagnetic interference (EMI) shielding: The increased electrical conductivity can be used for EMI shielding in electronic systems, protecting sensitive components from external electromagnetic radiation.
-
Automotive:
- Lightweight structural parts: The nanocomposites can be used to manufacture lightweight and strong components for vehicles, such as chassis, body panels, and interior parts, contributing to reduced weight, improved fuel efficiency, and lower emissions.
- Battery components: The improved electrical and thermal properties can be applied in the development of electric vehicle battery components, such as current collectors and thermal management systems, enhancing battery performance and safety.
-
EMI shielding and electrical connectors: The nanocomposites can be used for EMI shielding in electronic systems and as conductive materials for electrical connectors in vehicles.
-
Electronics:
- Flexible and wearable electronics: The nanocomposites can be employed in the fabrication of flexible and stretchable electronic devices, such as sensors, displays, and energy storage devices, due to their enhanced mechanical and electrical properties.
- Heat sinks and thermal interface materials: The improved thermal properties can be utilized in the development of heat sinks and thermal interface materials for efficient heat dissipation in electronic devices, prolonging their lifespan and performance.
-
Conductive adhesives and films: The nanocomposites can be used as conductive adhesives and films for electronic packaging and interconnects, providing reliable electrical connections and improved mechanical stability.
-
Energy:
- Solar cells: The enhanced electrical properties can be employed in the development of advanced solar cell components, such as transparent conductive electrodes, improving the overall efficiency of solar energy conversion.
-
Energy storage devices: The nanocomposites can be used as electrode materials or current collectors in batteries and supercapacitors, contributing to improved energy storage performance and safety.
-
Construction and Infrastructure:
- Reinforced composites: The nanocomposites can be incorporated into construction materials, such as concrete or fiber-reinforced plastics, to enhance their mechanical strength, durability, and resistance to environmental degradation.
- Smart materials and sensors: The improved electrical properties can be utilized in the development of smart materials and sensors for structural health monitoring, enabling real-time detection of damage or stress in buildings and infrastructure.
These are just a few examples of the potential real-world applications of the graphene-polymer nanocomposites with enhanced mechanical, thermal, and electrical properties. The versatility of these materials offers numerous opportunities for innovation and advancement across various industries.
Identify ethical concerns related to the discovery.
Several ethical concerns related to the discovery and application of graphene-polymer nanocomposites can be identified:
-
Environmental impact: The production and disposal of graphene-polymer nanocomposites may have potential environmental consequences, such as the release of nanoparticles into the environment, which could affect ecosystems and human health. It is essential to develop sustainable production methods and proper waste management strategies to minimize the environmental impact.
-
Health and safety: The potential health risks associated with the handling, processing, and use of graphene nanosheets and other nanomaterials need to be carefully assessed. Inhalation or ingestion of nanoparticles may pose health risks to workers involved in the production and application of these nanocomposites. Appropriate safety measures, such as personal protective equipment and proper ventilation, should be implemented to protect workers from exposure.
-
Resource allocation: The development and application of graphene-polymer nanocomposites may require significant financial and human resources. It is crucial to ensure that the allocation of resources for this research does not divert funding and attention away from other pressing societal needs, such as healthcare, education, and poverty alleviation.
-
Technology access and inequality: The benefits of graphene-polymer nanocomposites, such as improved performance and efficiency in various applications, may not be equally accessible to all individuals and communities. This could exacerbate existing inequalities and create new ones, particularly in developing countries where access to advanced materials and technologies may be limited. Efforts should be made to ensure that the benefits of these discoveries are distributed equitably and contribute to global sustainable development.
-
Regulation and standardization: The rapid development and application of graphene-polymer nanocomposites may outpace the establishment of appropriate regulations and standards to ensure their safe and responsible use. Policymakers, researchers, and industry stakeholders should collaborate to develop comprehensive guidelines and regulations that address potential risks and ethical concerns associated with these materials.
-
Public perception and acceptance: The public may have concerns about the safety and environmental impact of graphene-polymer nanocomposites, which could affect their acceptance and adoption. Transparent communication and engagement with the public are essential to address these concerns and build trust in the responsible development and application of these materials.
Addressing these ethical concerns is crucial to ensure the responsible development, application, and commercialization of graphene-polymer nanocomposites, as well as to maximize their potential benefits for society and the environment.
Suggest a collaboration with another field of research.
A promising collaboration for the development and application of graphene-polymer nanocomposites could be with the field of Biomedical Engineering. This interdisciplinary collaboration would combine the expertise of materials scientists and biomedical engineers to explore the potential of graphene-polymer nanocomposites in various healthcare applications, such as drug delivery, tissue engineering, and medical devices.
Potential collaborative research areas:
-
Drug delivery systems: Develop graphene-polymer nanocomposites as carriers for controlled drug release, targeting specific tissues or cells, and improving the efficacy and safety of therapeutic treatments. The unique properties of graphene, such as its large surface area and ability to adsorb and desorb molecules, could be harnessed to design advanced drug delivery systems.
-
Tissue engineering and regenerative medicine: Investigate the potential of graphene-polymer nanocomposites as scaffolds for tissue engineering, promoting cell adhesion, proliferation, and differentiation. The enhanced mechanical and electrical properties of these nanocomposites could be tailored to mimic the native extracellular matrix and support tissue regeneration.
-
Biosensors and diagnostics: Develop graphene-polymer nanocomposites as components of biosensors for the detection of biomolecules, such as proteins, nucleic acids, or small molecules, with high sensitivity and selectivity. The improved electrical properties of these nanocomposites could enable the development of rapid, accurate, and portable diagnostic tools for various medical applications.
-
Implantable medical devices: Explore the use of graphene-polymer nanocomposites in the fabrication of implantable medical devices, such as orthopedic implants, cardiovascular stents, or neural interfaces. The enhanced mechanical, thermal, and electrical properties of these nanocomposites could contribute to improved device performance, biocompatibility, and longevity.
-
Antimicrobial coatings and surfaces: Investigate the potential of graphene-polymer nanocomposites as antimicrobial coatings or surfaces for medical devices and equipment, reducing the risk of infection and promoting a sterile environment in healthcare settings.
This collaboration between materials science and biomedical engineering could lead to innovative solutions for various healthcare challenges, improving patient outcomes, and advancing the field of medical technology. By combining the expertise of both fields, researchers can explore the full potential of graphene-polymer nanocomposites in addressing unmet needs in healthcare and contribute to the development of novel, life-enhancing applications.
Propose a new interdisciplinary field based on the discovery.
Proposed Interdisciplinary Field: Nanostructured Functional Composites (NFC)
The discovery of graphene-polymer nanocomposites with enhanced mechanical, thermal, and electrical properties can lead to the establishment of a new interdisciplinary field called Nanostructured Functional Composites (NFC). This field would combine expertise from materials science, nanotechnology, physics, chemistry, and various engineering disciplines to design, synthesize, and characterize functional composites with tailored properties for a wide range of applications.
Key research areas in Nanostructured Functional Composites:
-
Nanofiller synthesis and functionalization: Develop novel methods for synthesizing and functionalizing various nanofillers, such as graphene, carbon nanotubes, nanowires, and nanoparticles, to enhance their compatibility with different polymer matrices and improve their dispersion within the composites.
-
Composite fabrication and processing: Investigate advanced fabrication and processing techniques, such as 3D printing, electrospinning, and in-situ polymerization, to create nanostructured functional composites with controlled architectures and optimized properties.
-
Structure-property relationships: Study the relationships between the composition, morphology, and dispersion of nanofillers within the polymer matrix and the resulting mechanical, thermal, electrical, and other functional properties of the composites.
-
Multifunctional composites: Design and develop composites with multiple functionalities, such as self-healing, self-sensing, or stimuli-responsive properties, by incorporating various nanofillers or functional additives into the polymer matrix.
-
Modeling and simulation: Employ computational methods, such as molecular dynamics simulations and finite element analysis, to predict and optimize the properties of nanostructured functional composites, guiding experimental efforts and reducing the need for extensive trial-and-error experimentation.
-
Sustainability and life cycle assessment: Evaluate the environmental impact, sustainability, and life cycle of nanostructured functional composites, aiming to develop eco-friendly materials and processes that minimize waste, energy consumption, and greenhouse gas emissions.
-
Application-specific development: Tailor the properties of nanostructured functional composites for specific applications in various industries, such as aerospace, automotive, electronics, energy, construction, and biomedical engineering, addressing unique challenges and requirements in each field.
The interdisciplinary field of Nanostructured Functional Composites (NFC) would bring together researchers from diverse backgrounds to advance the understanding, design, and application of nanostructured composites with tailored properties. By leveraging the unique properties of nanofillers and polymer matrices, this field could contribute to the development of innovative materials and technologies that address critical challenges in various industries and improve the quality of life.
Draft a press release explaining the findings to a general audience.
FOR IMMEDIATE RELEASE
Revolutionary Graphene-Polymer Nanocomposites Set to Transform Multiple Industries
[City, Date] - Researchers from [Institution] have made a groundbreaking discovery in the field of materials science, developing a new class of graphene-polymer nanocomposites with significantly enhanced mechanical, thermal, and electrical properties. This innovative material has the potential to revolutionize various industries, including aerospace, automotive, electronics, energy, and biomedical engineering.
Graphene, a single layer of carbon atoms arranged in a hexagonal lattice, is known for its exceptional strength, thermal conductivity, and electrical conductivity. By incorporating graphene nanosheets into polymer-based materials, the research team has successfully created lightweight, high-performance composites that outperform traditional materials in terms of strength, heat resistance, and electrical conductivity.
The researchers conducted a series of experiments to investigate the effects of adding different concentrations of graphene nanosheets to various polymer matrices. Their findings revealed a strong correlation between the concentration of graphene nanosheets and the resulting properties of the nanocomposites. The optimal concentration of graphene nanosheets led to significant improvements in the mechanical, thermal, and electrical properties of the composites, opening up new possibilities for their application in multiple industries.
Potential real-world applications of these graphene-polymer nanocomposites include lightweight structural components for aircraft and vehicles, advanced solar cell components, flexible and wearable electronics, and implantable medical devices. The versatility of these materials offers numerous opportunities for innovation and advancement across various sectors.
The research team is now exploring the potential of combining graphene nanosheets with other nanofillers, such as carbon nanotubes, to further enhance the performance of polymer-based nanocomposites. They are also collaborating with experts in biomedical engineering to investigate the use of these materials in drug delivery systems, tissue engineering, and biosensors.
This groundbreaking discovery marks a significant milestone in the field of materials science and has the potential to transform multiple industries by providing innovative solutions to current challenges. The development of graphene-polymer nanocomposites paves the way for a new generation of lightweight, high-performance materials that can improve the quality of life and contribute to a more sustainable future.
For more information about this research, please contact:
[Researcher's Name] [Title, Institution] [Email Address] [Phone Number]